Timothy Baker
The brief but brilliant career of Reginald Penrose (1921-1949; Queen’s, 1939) coincided with a ‘golden age’ of physics research at the Clarendon Laboratory. He was a pioneer with Brebis Bleaney in two new fields which developed into major branches of physics: microwave spectroscopy, and electron paramagnetic resonance. Penrose was poised for a distinguished career when he died unexpectedly in 1949 aged only 28.
In those days, scientists were known by their initials. In all his published papers, he was referred to as ‘R.P. Penrose’. When Bleaney died in 2007, the more circumspect obituarists stuck to these initials in describing their joint work. But some newspapers exercised ‘journalistic imagination’, and interpreted the name of Bleaney’s early collaborator as ‘Roger Penrose’. Even the Oxford Dictionary of National Biography’s entry on Bleaney, presumably taking its cue from the erring journalists, called him ‘Roger Penrose’ until it was corrected at my prompting in November 2022.
Being confused with a Nobel laureate is not an intrinsically bad fate (though Roger Penrose was only just starting as an undergraduate – at University College London – when Reginald Penrose died). But Reginald Penrose’s achievements – including in two pieces of work that were later considered for the Nobel Prize – stand on their own merits. Until the revised ODNB entry on Bleaney, Penrose’s correct Christian name had not appeared in print since the obituary that Bleaney published in Nature in 1949. Nearly three-quarters of a century on, it was high time that Reginald Penrose emerged from the shadows of history.
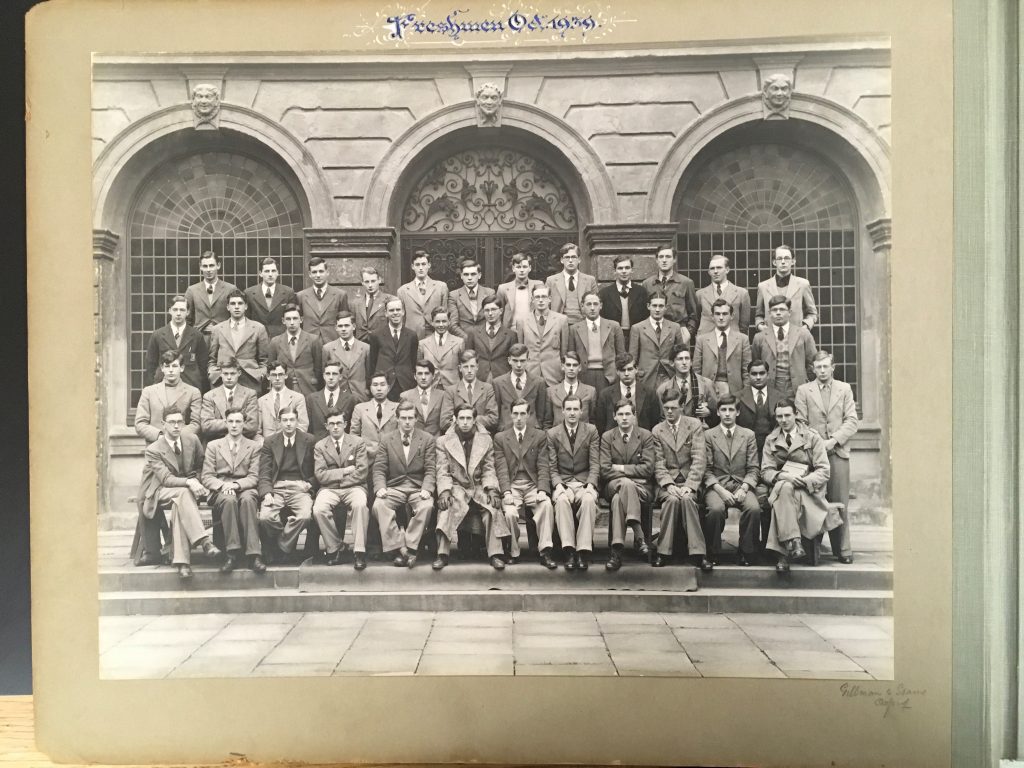
Reginald Percy Penrose was born on 6th January 1921 at Salford, Lancashire. He was educated at Altrincham Grammar School, Cheshire. He came up to Queen’s College in 1939 to read physics. Because of the war, his course was shortened to two years. So in 1941, remaining at Queen’s for his DPhil, he joined the Admiralty research group at the Clarendon Laboratory, which was working on magnetrons and klystrons as transmitters and receivers for centimetric-wavelength radar. His supervisor was Arthur Cooke, of New College, but he also worked closely with Brebis Bleaney, of St John’s.
Centimetric radar was a vital weapon of war, since the wavelength was short enough to detect U-boats’ conning towers in the wastes of the Atlantic. Because of the small size of these targets, the beam needed to be powerful so that its intrinsically weaker reflection could be detected. The prototype 10-centimetre cavity magnetron transmitter, invented at Birmingham University in 1940 by John Randall and Harry Boot, and taken to the United States for mass production, along with improved klystron transmitters developed at Oxford, was described by an American commentator as ‘the most valuable cargo ever to cross the Atlantic’. Centimetric microwave radar helped to turn the tide in the Battle of the Atlantic, assisted by the ‘transmit-receive’ switch, which Arthur Cooke invented in 1940. This switch enabled the same aerial to be used alternately for pulsed transmission and for reception of reflections, so that for the first time it was possible to instal radar in aircraft, which could thus attack submarines directly on the surface.
Reginald Penrose obtained his DPhil in 1946. Because of its military application, his thesis, Measurements on high frequency electromagnetic oscillations, now in the Bodleian Library, was originally stamped ‘Secret’. Because of the need to maximise the power of the transmitted radar pulses and their reflections, an important element of the Clarendon’s wartime research concerned measurement of the absorption of microwaves by various materials. Different substances absorb radiation at different wavelengths, because only photons of precise frequencies, and thus with precise quanta of energy, can be absorbed by their electrons to allow them to ‘jump’ between physically permitted energy levels in the atom. The phenomenon is familiar from the dark ‘Fraunhofer lines’ in the visible spectrum. In the microwave spectrum, water is an absorber (that is how microwave ovens work), so Arthur Cooke’s transmit-receive switch used water vapour to shield the receiver from the powerful transmitting pulses. Penrose’s DPhil research concerned the power characteristics of transmitters, and the absorption characteristics of solid dielectric insulators and of another gas: ammonia.
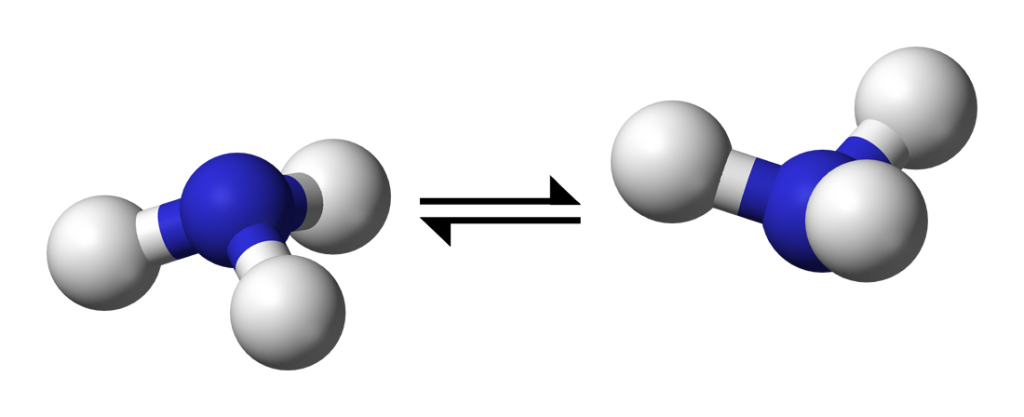
Penrose continued at Queen’s for post-doctoral research, and continued to work with Bleaney and others, including Bleaney’s future wife Betty Plumpton, as they ‘beat swords into plowshares’, using war-surplus radar equipment for fundamental research in microwave spectroscopy.
Bleaney and Penrose first turned their attention to ammonia. The ammonia molecule consists of a triangle of hydrogen atoms, bonded tetrahedrally to a nitrogen atom. Absorbing a quantum of microwave radiation can make the nitrogen atom ‘flip’ from one side to the other of the hydrogen triangle. The absorption frequency gives rise to an ‘inversion spectrum’. The ability of the bonding electrons to ‘jump’ between different energy states gives rise to a ‘fine structure’ in the spectrum, with different absorption frequencies corresponding to different ‘jumps’. In 1945 Bleaney and Penrose were the first to discover this ‘fine structure’ in the ammonia spectrum.
Though they did not take this research much further, it had momentous consequences. In America Charles Townes was similarly using war surplus equipment to investigate ammonia’s inversion spectrum. He independently found the ‘fine structure’, but yielded priority to Bleaney and Penrose. Unlike the Oxford team, Townes did however continue in the field, and mapped the spectrum in detail. This work led on to the discovery that electrons in ammonia could be stimulated into long-lasting energy states, and so to Townes’s invention of the Maser (‘Microwave Amplification by Stimulated Emission of Radiation’), the first powerful source of coherent light. Further research by others led on to the invention of the Maser’s equivalent in the visible spectrum, the Laser (Light Amplification by Stimulated Emission of Radiation), one of the fundamental technologies of the modern world.
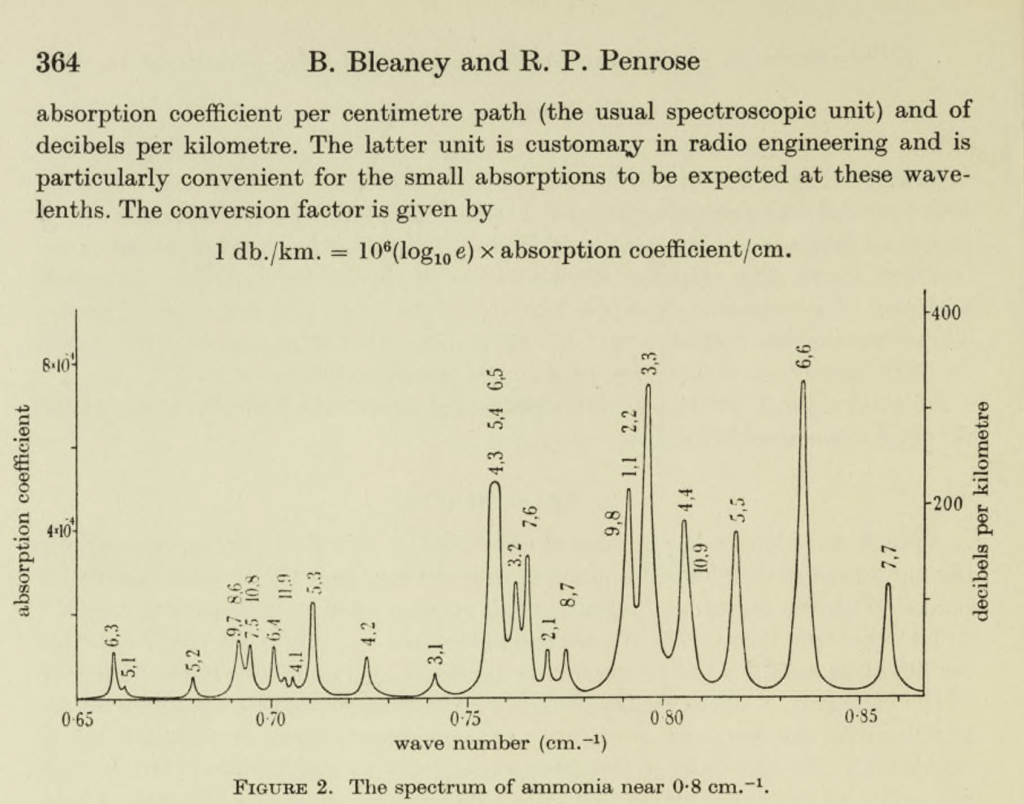
Townes recalled in How the Laser Happened: Adventures of a Scientist that, probably in the late 1950s, when Bleaney was nominated for it, this field was being considered for the Nobel Prize in Physics. Townes, later a Nobel laureate himself, pointed Bleaney’s priority out to the Nobel Committee; he suggested in his book that, though Bleaney was the discoverer, the fact that it was Townes who carried research forward might have prevented the Nobel Committee from coming to a clear conclusion about the appropriate recipient, so they awarded that Prize to another field. This was the first ‘Nobel near-miss’ to which Reginald Penrose contributed.
Bleaney and Penrose turned to another field in which the former had been one of the pioneers: electron paramagnetic resonance (EPR), which chemists call electron spin resonance. Once again using war surplus radar equipment, Bleaney discovered EPR in 1945. Unknown to him, at Kazan in Russia Yevgeny Zavoisky had already found this phenomenon in 1944. This time, however, it was Zavoisky who did not follow up his discovery; and Bleaney, initially with Penrose’s and Plumpton’s help, who carried the work forward, and turned Oxford into the leading EPR research school in the world.
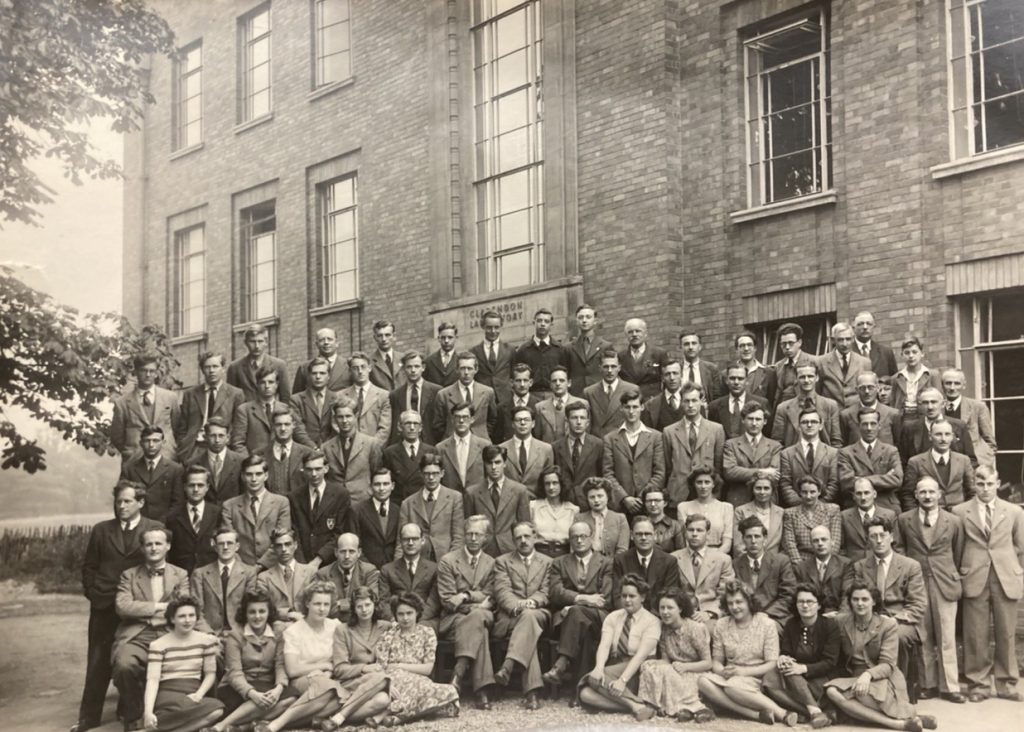
Electrons have a quantum property called ‘spin’ which makes them behave like tiny magnets. Paramagnetic materials have unpaired electrons in their atoms. So when an external magnetic field is applied, the unpaired electrons align with it, either parallel or antiparallel to the field, and the material becomes magnetic. The two alignments have different energy levels. Microwaves of the right ‘resonant’ frequency can make the electron absorb a photon and ‘flip’ from the lower to the higher energy level, causing a line in the spectrum. The electron’s interaction with the changing magnetic field caused by its own orbital motion within the atom causes this line to split, creating the ‘fine structure’ of the spectrum.
EPR spectroscopy is used to investigate paramagnetic substances whose atoms are ‘diluted’ in the lattice of a non-magnetic crystal, so that interference with other atoms is minimised. The sample was reduced to low temperature, close to Absolute Zero, to limit unwanted thermal vibration. A strong external magnetic field was applied, aligning the unpaired electrons in the two spin states, so when microwave radiation of the right frequency was shone through the sample, it could be absorbed by the electrons to ‘flip’ them between states. Each element has a characteristic electron structure, so the spectrum could be used to characterise the material. As in the case of ammonia, each element has a characteristic ‘fine structure’ corresponding to ‘jumps’ between a range of physically permitted energy levels. In the late 1940s Bleaney and Penrose developed this new technique into a powerful tool for measuring and investigating the magnetic properties of atoms and materials.
In 1948 Reginald Penrose was appointed to a lectureship at Leeds University. Before moving there, however, he went for a six-month posting to the Kamerlingh Onnes Laboratory at Leiden. Here he continued EPR research and, while analysing the EPR spectrum of a crystal of copper potassium sulphate, was the first to observe the ‘hyperfine structure’ in the electron transition, which results from the interaction between the electron and the atomic nucleus. Once again, this was the first step in a wide field of research in the magnetic spectroscopy of solids, which has resulted in many technological applications. My father, Michael Baker, who succeeded Penrose as a pupil and graduate student of Bleaney from 1948, recalled the excitement of discussing these new results in about 1950: ‘My lifelong association with hyperfine structure started with Brebis telling me in one tutorial about the six-line hyperfine structure of 55 Manganese; this must have been soon after Penrose published the first article on hyperfine structure in diluted paramagnetic salts, that of 63, 65 Copper in 1949’. The ‘hyperfine structure’ became a main focus of EPR research, and enabled the development of electron-nuclear double resonance (ENDOR), in which EPR and NMR are applied simultaneously to the same sample. ENDOR is able to amplify the nuclear signal, and therefore greatly expanded the scope of nuclear magnetic research.
In 1966, along with Zavoisky, Bleaney was nominated again for the Nobel Prize in Physics. This nomination must have been for the invention and exploitation of EPR spectroscopy. My father, who also considered Bleaney’s work on EPR worthy of the Nobel Prize, thought that the field might have been crowded out by NMR research, for which the Prize was awarded twice. It is good to know that Bleaney was at any rate considered for it. This is thus the second ‘Nobel near-miss’ that Reginald Penrose was involved with.
Publication of the ‘hyperfine’ result was delayed because Penrose became ill with a brain tumour in early 1949. Surgery appeared to have been successful, but shortly afterwards Penrose suddenly and unexpectedly died in hospital at Wassenaar, near Leiden, on 28 April 1949, aged only 28. So his last and greatest discovery was announced posthumously.
There is a poignancy in the obituary that Bleaney wrote for Nature, and the note that C. J. Gorter added to his write-up of Penrose’s laboratory notes for the same edition, on 25th June 1949. Bleaney wrote: ‘His life centred in his research, … and his enthusiasm in following up a new idea led him to make much of the apparatus himself, for he was a skilful machinist. A cheerful companion, he was liked by all; I was particularly fortunate in enjoying four years of close partnership with him in the Clarendon Laboratory. Our intimate relationship did not cease when he left Oxford, and the exchange of results and ideas with him will be greatly missed. His death at the age of 28 deprives microwave physics of one of its exponents, and his scientific papers (some of them yet to appear) form the memorial to his pioneer work in this field.’
Bleaney continued to research EPR for another eight years, until he succeeded as Dr Lee’s Professor of Experimental Philosophy in 1957. At this stage my father took over Bleaney’s research group. As a pioneer of ENDOR, and as the initiator of what has become an extensive field of scientific and technological innovation, EPR and ENDOR of impurities in diamond, Michael Baker’s career gives some idea of what Reginald Penrose might have gone on to achieve had he lived.
Let Reginald Penrose no longer be the ‘forgotten physicist’.